النبات
مواضيع عامة في علم النبات
الجذور - السيقان - الأوراق
النباتات الوعائية واللاوعائية
البذور (مغطاة البذور - عاريات البذور)
الطحالب
النباتات الطبية
الحيوان
مواضيع عامة في علم الحيوان
علم التشريح
التنوع الإحيائي
البايلوجيا الخلوية
الأحياء المجهرية
البكتيريا
الفطريات
الطفيليات
الفايروسات
علم الأمراض
الاورام
الامراض الوراثية
الامراض المناعية
الامراض المدارية
اضطرابات الدورة الدموية
مواضيع عامة في علم الامراض
الحشرات
التقانة الإحيائية
مواضيع عامة في التقانة الإحيائية
التقنية الحيوية المكروبية
التقنية الحيوية والميكروبات
الفعاليات الحيوية
وراثة الاحياء المجهرية
تصنيف الاحياء المجهرية
الاحياء المجهرية في الطبيعة
أيض الاجهاد
التقنية الحيوية والبيئة
التقنية الحيوية والطب
التقنية الحيوية والزراعة
التقنية الحيوية والصناعة
التقنية الحيوية والطاقة
البحار والطحالب الصغيرة
عزل البروتين
هندسة الجينات
التقنية الحياتية النانوية
مفاهيم التقنية الحيوية النانوية
التراكيب النانوية والمجاهر المستخدمة في رؤيتها
تصنيع وتخليق المواد النانوية
تطبيقات التقنية النانوية والحيوية النانوية
الرقائق والمتحسسات الحيوية
المصفوفات المجهرية وحاسوب الدنا
اللقاحات
البيئة والتلوث
علم الأجنة
اعضاء التكاثر وتشكل الاعراس
الاخصاب
التشطر
العصيبة وتشكل الجسيدات
تشكل اللواحق الجنينية
تكون المعيدة وظهور الطبقات الجنينية
مقدمة لعلم الاجنة
الأحياء الجزيئي
مواضيع عامة في الاحياء الجزيئي
علم وظائف الأعضاء
الغدد
مواضيع عامة في الغدد
الغدد الصم و هرموناتها
الجسم تحت السريري
الغدة النخامية
الغدة الكظرية
الغدة التناسلية
الغدة الدرقية والجار الدرقية
الغدة البنكرياسية
الغدة الصنوبرية
مواضيع عامة في علم وظائف الاعضاء
الخلية الحيوانية
الجهاز العصبي
أعضاء الحس
الجهاز العضلي
السوائل الجسمية
الجهاز الدوري والليمف
الجهاز التنفسي
الجهاز الهضمي
الجهاز البولي
المضادات الحيوية
مواضيع عامة في المضادات الحيوية
مضادات البكتيريا
مضادات الفطريات
مضادات الطفيليات
مضادات الفايروسات
علم الخلية
الوراثة
الأحياء العامة
المناعة
التحليلات المرضية
الكيمياء الحيوية
مواضيع متنوعة أخرى
الانزيمات
Hydrophobic Chromatography
المؤلف:
S. Shaltiel
المصدر:
Methods Enzymol. 104, 69.
الجزء والصفحة:
23-5-2016
3227
Hydrophobic Chromatography
1. The Basic Principle
Hydrophobic chromatography (HC) is a chromatographic method for the characterization, separation, and isolation of proteins and cells (1). It is based on hydrophobic (water-repelling( interactions between (a) hydrocarbon chains of different lengths and shapes that are anchored on inert beads and (b) the hydrophobic “pockets” or “patches” that are found on the accessible surface of proteins. Such hydrocarbon chains interact with the protein molecules submerged in water and bind them to minimize the surface of the hydrophobic area that is exposed to the water. This binding brings about the release of “ordered” water molecules, which forms the basis for the hydrophobic interactions. It turns out that proteins can be distinguished and resolved from one another by HC, because they differ in the size, number, and water repellence of their hydrophobic “patches” or “pockets.” This basic principle in chromatography (2, 3) is also known as hydrophobic interaction chromatography and reversed-phase high-performance liquid chromatography ( HPLC). It has now become a very powerful method of separation and has been used for research in biochemistry, molecular biology, and biotechnology—for example, in the preparation and characterization of drugs such as enzymes, hormones, antibodies, and receptors.
2. The Development of HC
HC was developed as a result of an unexpected observation made while attempting to make use of affinity chromatography (AC) (4). The basic concept in AC is based on the use of the specific affinity between the biorecognition site of a protein (an enzyme, an antibody, a receptor, etc.) and one of its biospecific ligands (a substrate, an inhibitor, a hormone, or a regulatory metabolite) that is covalently linked to an inert matrix backbone and can be regarded as an immobilized “bait” for the protein. The column with the immobilized bait is then used to “fish out” the desired protein from a mixture. While developing the basic principles of AC, it was observed that the purification of a desired protein is often improved by interposing a hydrocarbon chain (an “arm”) between the ligand and the matrix backbone (4, 5). It was presumed that such an arm relieves the steric restrictions imposed by the backbone on the ligand, thereby increasing its flexibility as well as its availability to the protein (5, 6). It was also implied that the hydrocarbon arms do not alter the inert nature of the matrix, a condition that obviously has to be ensured to preserve a strictly biospecific (active-site mediated) adsorption of the extracted protein. This assumption seemed reasonable at the time (at least with water-soluble proteins), because it had been shown in the case of lysozyme that “most of the markedly nonpolar and hydrophobic side chains ¼ are shielded from the surrounding liquid by more polar parts of the molecule” and that, as predicted by Sir Eric Rideal and Irving Langmuir, “lysozyme is quite well described as an oil drop with a polar coat” (7).
For the purification of enzymes involved in glycogen metabolism, glycogen (the “bait”) was anchored onto beaded agarose . Unexpectedly, two glycogen-coated agarose preparations that differed only in the length of the hydrocarbon chains bridging the ligand and the agarose bead (Seph-C8-glycogen and Seph-C4-glycogen) exhibited different behaviors: Whereas the column material with eight-carbon-atom bridges adsorbed glycogen phosphorylase b, that with four-carbon-atom bridges did not even retard it (2). Control experiments revealed that both the adsorption of the enzyme by Seph-C8-glycogen and its exclusion by Seph-C 4-glycogen could be reproduced with columns that contained no glycogen whatsoever (Seph-C8-NH 2 and Seph-C4-NH 2), indicating that free w-aminooctyl chains (which had not reacted with glycogen) were mainly responsible for the retention of phosphorylase on Seph-C8-glycogen and also raising the possibility that the very length of the hydrocarbon chain determines the retention power of these columns.
3. Homologous Series of Alkylagarose Columns
To challenge this working hypothesis, a homologous series of alkylagarose columns was synthesized, and their retention power was tested with two enzymes, glycogen phosphorylase b and glyceraldehyde-3-phosphate dehydrogenase (Fig. 1). This “homologous series of alkylagarose columns” (Seph-Cn) refers to a set of columns that are identical in their ligand density, net charge, and ultrastructure. Such a set of columns can be considered as a series of consecutive controls in which each column differs from the preceding one in the series only in having hydrocarbon chains one carbon atom longer. In general, under the same experimental conditions, different proteins display different adsorption profiles on the Seph-Cn columns (1-3). For example, under the conditions used to retain phosphorylase b (n≥4), D-glyceraldehyde-3-phosphate dehydrogenase was not even retarded on any of the columns tested ( n = 14). Thus, while Seph-C1 and Seph-C2 would not distinguish between the two enzymes and would exclude both of them, Seph-C3 would begin to resolve them, and Seph-C4 would separate them efficiently, indicating that the ability to bind and to discriminate between two proteins (ie, to resolve and separate them) is a function of the number of carbon atoms in the hydrocarbon chains of the columns (Fig. 1). In addition, the above results showed that it is possible to adjust the tightness of adsorption of a given protein and avoid an overly strong retention, which would then require drastic conditions for elution that might denature the eluted protein. Finally, these studies illustrated the use of “deforming agents” (local, fully reversible denaturants that loosen the structure of the proteins) as efficient and delicate eluents in detaching adsorbed proteins from HC columns.
Figure 1. Preferential adsorption of glycogen phosphorylase b on alkylagarose columns .
HC provides now a general, systematic approach to the purification of water-soluble, as well as lipophilic, proteins. This approach makes use of homologous series of alkylagaroses and their derivatives (Seph-Cn-X where X = H, NH2, COOH, OH, C 6H5, etc.; Fig. 2) to achieve resolution and purification of proteins and cells. Each member in these series offers flexible hydrophobic arms or “yardsticks” that interact with accessible hydrophobic “patches” or “pockets” in the various proteins, retaining only some proteins out of a mixture. Further resolution is then achieved by gradually changing the nature of the eluent. The wide applicability of this type of chromatography in protein purification and in separating cells is now well accepted. Procedures for the synthesis and the characterization of Seph-Cn ( n = 112) are described in Ref. 1.
Figure 2. Examples of homologous series of alkylagarose derivatives (Seph-Cn-X) that can be used for HC (1).
4. Selecting a Column for a Given Purification—the Exploratory Kit
The selection of a suitable substituted agarose within a given homologous series (eg, Seph-C n) is achieved by means of an exploratory group of column materials designed for this purpose (1). Each column in the kit contains one member of the series. If, under certain conditions, a specific protein is adsorbed onto hydrocarbon chains X carbon atoms long, and if a change in the eluent promotes exclusion from Seph-Cx, then this is the column of choice. An example of an adsorption-elution profile of glycogen phosphorylase, b, on the Seph-Cn series, is given in Figure 3a. As seen in this figure, as the length of the hydrocarbon chains increases, less protein is excluded from the column. While Seph-C0 and Seph-C1 exclude all the protein, Seph-C 2 and Seph-C3 retard it, whereas Seph-C4 and higher members of the series retain the protein. By passage of a 0.4 M imidazolium-citrate buffer, a “deformer” for glycogen phosphorylase b (8, 9), it is possible to elute the enzyme quantitatively from the Seph-C4 column, but not from higher members in the series. In fact, recovery of the enzyme from Seph-C 6 was achieved only with a drastic eluent, 0.2 M CH3COOH, which displaces the enzyme but also denatures it. On the basis of such results, Seph-C4 should be chosen for the preparative purification of this enzyme; and indeed this column allowed a 60- to 100-fold purification in one step, with >95% recovery of enzyme activity (Fig. 3b).
Figure 3. Hydrophobic chromatography (a) Adsorption profile of glycogen phosphorylase b on a Seph-Cn kit. The filled circles indicate the amount of protein passing straight through the column. Empty circles indicate the amount of protein eluted by a “deforming buffer.” (b) Preparative purification of the enzyme from a muscle extract. Absorbance
(empty circles) and specific activity (filled circles) were monitored. Nonadsorbed protein was removed by washing, and elution with the “deforming buffer” was initiated at the fraction indicated by the arrow (1, 2).
5. Means of Elution
Studies aimed at the optimization of elution from alkylagaroses have shown that proteins can be desorbed from such columns by a variety of means: polarity-reducing agents, specific deformers, mild detergents, low concentration of denaturants, alteration in pH or temperature, and changes in ionic strength and ionic composition. Because the availability of hydrophobic crevices or patches on the surface of a protein appears to depend on its conformation, and because the retention of proteins by alkylagaroses depends largely on the lipophilicity, size, shape, and number of these crevices and patches, the above-mentioned means of elution may function either by directly disrupting the hydrophobic interactions between the column material and the protein or by changing the conformation of the protein. In fact, both mechanisms may often operate simultaneously. High selectivity in elution may also be achieved by using biospecific ligands, such as coenzymes, substrates, specific metal ions, and allosteric effectors, which often bring about ligand-induced conformational changes in proteins.
6. Useful Features of Hydrophobic Chromatography
The important features of hydrophobic chromatography are as follows: (i) It provides an independent criterion for the resolution of macromolecules. (ii) It provides means for gradually adjusting the adsorption forces and thereby avoiding an overly strong retention. (iii) It can be used for the detection of conformational changes in proteins when these are reflected in the size or distribution of hydrophobic crevices or patches. A potential use of these column materials may be in the purification and study of lipophilic membrane-bound proteins that have accessible hydrophobic regions used for their localization within the membrane. (iv) It provides a basis for the development of automatic consecutive fractionators (1) for the purification of proteins.
7. On the Mechanism of Action of Alkylagaroses
In their functional conformation, water-soluble proteins are folded so as to “bury” as many as possible of their hydrophobic side chains in the interior of the molecule and to expose as many as possible of their polar, charged side chains to interaction with water. It is now clear, however, that complete burying of all hydrophobic groups is generally not achieved, leaving some hydrophobic groups exposed at the surface of the protein. Together with hydrophobic components of charged amino acids, such as the (-CH2-), stretches of lysine and glutamic acid residues, or the phenyl ring of tyrosine residues, these form hydrophobic “patches” or “pockets” at the surface of the molecule (Fig. 4). A sufficiently large hydrophobic patch may constitute a binding site for the hydrocarbon chains implanted on the hydrophilic agarose matrix and form “hydrophobic bonds,” freeing “ordered” water molecules and allowing them to interact with each other (10-12). The available hydrophobic patches and pockets of different proteins vary in number, size, shape, and lipophilicity, and these variations are reflected in the relative affinities of different proteins for a specific alkylagarose. It is the properties of such patches, and perhaps their distribution on the surface of different proteins, that play a major role in the resolution of proteins on alkylagaroses.
Figure 4. Schematic representation of the surface of a protein molecule with an exposed hydrophobic amino acid residue (tryptophan) and hydrophobic “pockets” or “patches” (hatched). The scheme also illustrates how hydrophobic constituents of several nonhydrophobic amino acids bearing hydrophobic functional groups can together form a site capable of accommodating a hydrophobic hydrocarbon chain (1).
References
1. S. Shaltiel (1984) Methods Enzymol. 104, 69.
2. Z. Er-el, Y. Zaidenzaig, and S. Shaltiel (1972) Biochem. Biophys. Res. Commun. 49, 383.
3. S. Shaltiel and Z. Er-el (1973) Proc. Natl. Acad. Sci. USA 70, 778.
4. P. Cuatrecasas, M. Wilchek, and C. B. Anfinsen (1968) Proc. Natl. Acad. Sci. USA 61, 636.
5. P. Cuatrecasas (1970) J. Biol. Chem. 245, 3059.
6. P. Cuatrecasas and C. B. Anfinsen (1971) Annu. Rev. Biochem. 40, 259.
7. D. C. Phillips (1966) Sci. Am. November, 78.
8. S. Shaltiel, J. L. Hedrick, and E. H. Fischer (1966) Biochemistry 5, 2108.
9. J. L. Hedrick, S. Shaltiel, and E. H. Fischer (1969) Biochemistry 8, 2422.
10. G. S. Hartley (1936) Aqueous Solutions of Paraffin-Chain Salts Hermann, Paris.
11. H. S. Frank and M. W. Evans (1945) J. Chem. Phys. 13, 507.
12. W. Kauzmann (1959) Adv. Protein Chem. 14, 1.
الاكثر قراءة في مواضيع عامة في الاحياء الجزيئي
اخر الاخبار
اخبار العتبة العباسية المقدسة
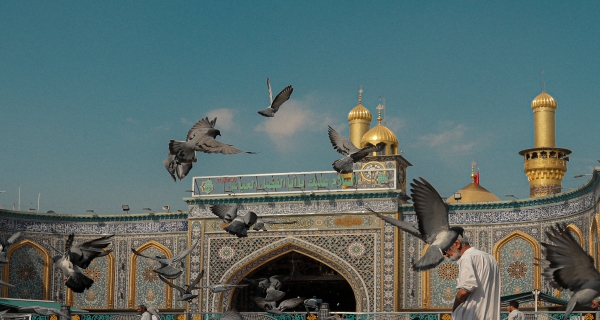
الآخبار الصحية
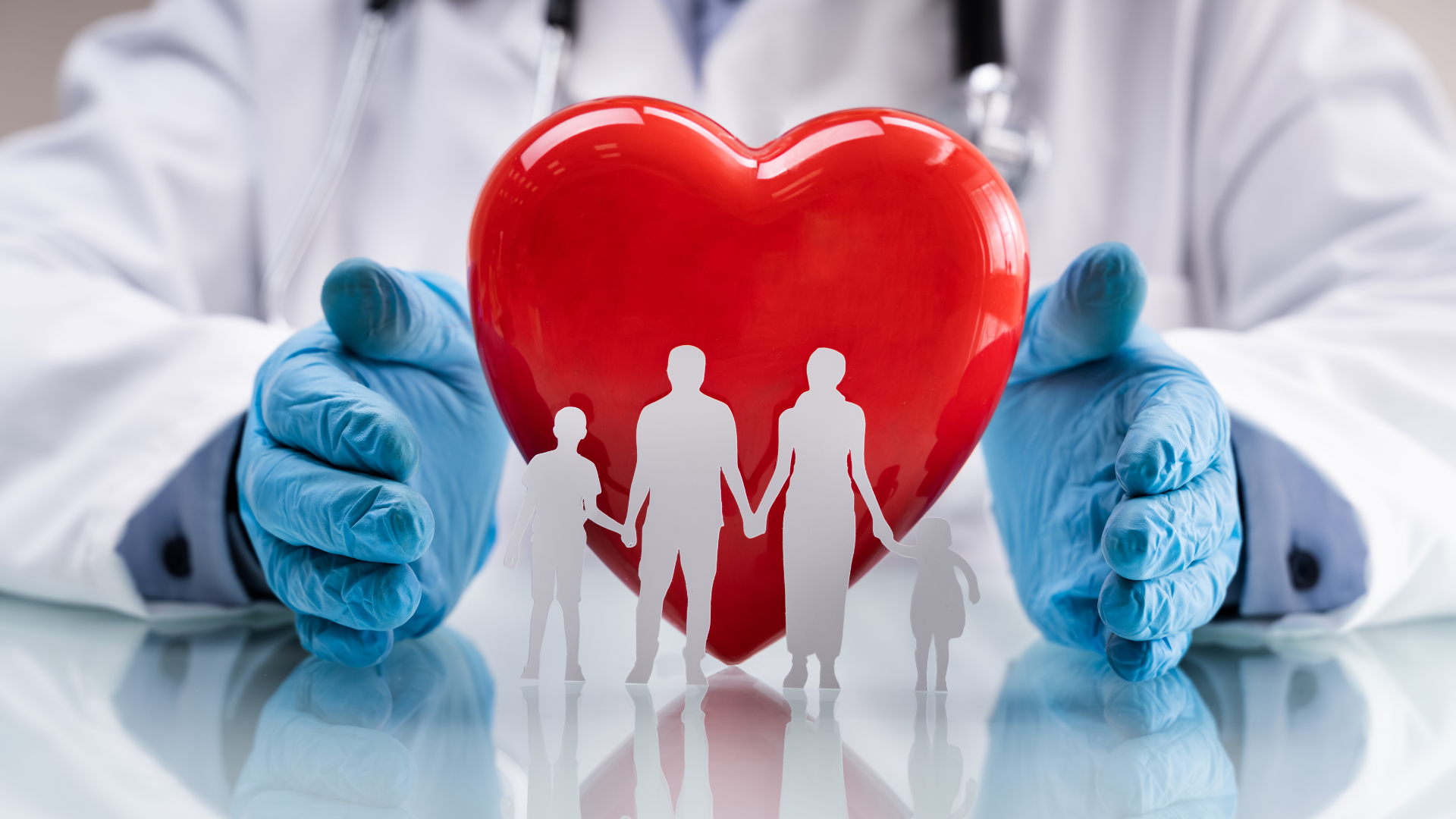